Category: PU Technology
PU Technology
The Power of Metal Catalysts: Unveiling their Role in Chemical Reactions and Industrial Applications
Introduction
Metal catalysts have been a cornerstone of chemical reactions and industrial processes for centuries. These powerful agents facilitate and accelerate various chemical transformations, making them indispensable in numerous sectors, including pharmaceuticals, agriculture, energy, and environmental protection. This article aims to delve into the fascinating world of metal catalysts, exploring their properties, functions, and applications, as well as the challenges and opportunities they present in modern science and industry.
What are Metal Catalysts?
Metal catalysts are substances that increase the rate of chemical reactions without being consumed in the process. They are typically composed of transition metals, such as platinum, palladium, nickel, and iron, which possess unique electronic configurations that allow them to interact with reactants and lower the activation energy required for a reaction to occur. This interaction facilitates the formation of new bonds and the breaking of old ones, ultimately leading to the desired products.
Properties and Functions of Metal Catalysts
Metal catalysts exhibit several essential properties that make them highly effective in promoting chemical reactions. These include:
-
High surface area: Metal catalysts often have a large surface area, which allows for greater contact between the catalyst and reactants, thereby increasing the reaction rate.
-
Active sites: The surface of metal catalysts contains active sites, which are specific locations where reactant molecules can bind and undergo chemical transformations.
-
Selectivity: Metal catalysts can exhibit high selectivity, meaning they favor the formation of specific products over others. This property is crucial in industrial applications, where the production of a single desired product is often the primary goal.
-
Stability: Metal catalysts must be stable under the reaction conditions to maintain their activity and prevent degradation.
-
Recyclability: In many cases, metal catalysts can be recovered and reused, making them more cost-effective and environmentally friendly.
Applications of Metal Catalysts
Metal catalysts play a crucial role in a wide range of industrial processes and chemical reactions. Some notable applications include:
-
Pharmaceuticals: Metal catalysts are extensively used in the synthesis of pharmaceutical compounds, enabling the production of life-saving drugs and medicines. For example, palladium catalysts are often employed in cross-coupling reactions, which allow for the formation of complex carbon-carbon bonds found in many pharmaceutical agents.
-
Agriculture: The production of fertilizers, such as ammonia, relies on metal catalysts to facilitate the conversion of nitrogen and hydrogen gases into ammonia. This process, known as the Haber-Bosch process, utilizes iron-based catalysts and has revolutionized agriculture by providing a reliable source of nitrogen-rich fertilizers.
-
Energy: Metal catalysts are essential in various energy-related processes, such as the refining of crude oil, the production of biodiesel, and the generation of hydrogen through steam reforming. For instance, platinum and nickel catalysts are commonly used in fuel cells to promote the conversion of hydrogen and oxygen into water, generating electricity in the process.
-
Environmental protection: Metal catalysts play a vital role in reducing pollutants and greenhouse gas emissions. For example, automotive catalytic converters use platinum, palladium, and rhodium catalysts to convert harmful exhaust gases, such as carbon monoxide, nitrogen oxides, and hydrocarbons, into less harmful substances like carbon dioxide, nitrogen, and water vapor.
Challenges and Opportunities
Despite their numerous benefits, metal catalysts also present several challenges that must be addressed to ensure their continued use and development. These challenges include:
-
Cost: Many metal catalysts are derived from precious metals, which can be expensive and subject to price fluctuations. Developing alternative, more cost-effective catalysts is an area of active research.
-
Scarcity: Some metal catalysts, such as platinum and palladium, are relatively rare and may become depleted over time. Efforts are underway to identify and develop new catalysts based on more abundant metals.
-
Deactivation: Metal catalysts can lose their activity over time due to various factors, such as poisoning, sintering, or mechanical degradation. Enhancing the stability and longevity of metal catalysts is an important area of research.
-
Environmental impact: While metal catalysts can help reduce pollutants and greenhouse gas emissions, their production, use, and disposal can also have negative environmental consequences. Developing more sustainable and eco-friendly catalysts is a critical goal for the future.
In conclusion, metal catalysts are powerful agents that play a crucial role in various chemical reactions and industrial processes. Their unique properties and functions make them indispensable in sectors such as pharmaceuticals, agriculture, energy, and environmental protection. However, challenges related to cost, scarcity, deactivation, and environmental impact must be addressed to ensure the continued development and use of these vital materials. Through ongoing research and innovation, metal catalysts will undoubtedly continue to shape the future of science and industry, unlocking new possibilities and driving progress in countless applications.
Recommended Reading:
cyclohexylamine
Tetrachloroethylene Perchloroethylene CAS:127-18-4
DABCO MP608/Delayed equilibrium catalyst
NT CAT DMDEE
NT CAT PC-5
NT CAT DMP-30
N-Acetylmorpholine
N-Ethylmorpholine
Morpholine
High Quality 3164-85-0 / K-15 Catalyst / Potassium Isooctanoate
Commonly used products in the field of polyurethane foam
Application areas: polyurethane rigid foam, flexible foam, self-skinning, shoe materials
Characteristics: delayed, heat-sensitive
Function: prolonging emulsion time, prolonging drawing time
Use system: MDI/TDI/PAPI/mixed isocyanate
DY-215
Special amine delayed gel catalyst, providing excellent pre-flow, delaying drawing time, not affecting post-curing, accelerating product release.
DY-225
Diol solution of modified bis(dimethylaminoethyl) ether, delayed foaming catalyst, delayed emulsification time, improve fluidity, shorten demoulding time.
DY-300
Delayed gel catalyst, improve fluidity, good open porosity. Used for moulding high resilience, self-skinning, etc.
DY-400
Delayed foaming catalyst, can improve the carrying capacity of foam. Used in moulding high resilience, self-skinning, microcellular foaming, etc.
DY-8154
Modified triethylene diamine diol solution, gel catalyst with delayed action, can improve fluidity and shorten demoulding time.
Commonly used products in the field of polyurethane CASE
Application areas: polyurethane coatings, adhesives, elastomers, polyurethane acrylic coatings, fabric coatings, polyurethane pastes, leather finishes, composites, etc.
Characteristics: Delayed, heat-sensitive
Function: Extend the open time, delay the rise of viscosity, does not affect the post-curing
Use system: MDI/TDI/IPDI/HDI/H12MDI/ADI/XDI/mixed isocyanate
Delayed catalyst effect picture Introduction: In CASE field (adhesives, coatings, elastomers), the most commonly used catalyst is DY-12 (dibutyltin dilaurate), which has good selectivity to -NCO and -OH and high activity, but in the preparation of complex products, the operation time given to the workers is short, and it can’t satisfy the construction demand, in this condition, Shanghai Deyin Chemical Co. Ltd. has developed a series of delayed catalysts to recommend more suitable products for different isocyanate systems and construction conditions.
DY-5115
Used in polyurethane adhesives, coatings, elastomers, composites and other fields.
DY-5300
Used in polyurethane adhesives, coatings, elastomers, composites, etc.
DY-5320
Used in polyurethane elastomers, adhesives, sealants, elastic paints, leather finishing agents and other industries.
DY-5558
Used in one-component polyurethane adhesives, coatings, leather finishing agents and other industries
DY-5503
For polyurethane elastomers, adhesives, sealants, elastic paints, leather finishing agents and other industries
DY-5508
For polyurethane elastomers, adhesives, sealants, elastic paints, leather finishing agents and other industries
DY-5980
Used in polyurethane adhesives, leather finishing agents, coatings, microporous elastomers, etc.
DY-5982
Used in polyurethane adhesives, coatings, microporous elastomers, etc.
Recommended reading:
Addocat 106/TEDA-L33B/DABCO POLYCAT
Non-emissive polyurethane catalyst/Dabco NE1060 catalyst
NT CAT U26
NT CAT DMEA
High Quality N-(dimethylaminopropyl)diisopropanolamine / CAS 63469-23-8 / N-(3-dimethyl amino propyl)-N N-diisopropanolamine
Toyocat ETS Foaming catalyst Tosoh
BDMAEE Exporter
Jeffcat ZF-22
4-Acryloylmorpholine
NT CAT TMR-2
Hard Foam Catalysts: A Comprehensive Overview
Introduction
Hard foam catalysts have emerged as a significant development in the field of chemical engineering and industrial applications. These unique materials combine the advantages of traditional catalysts with the benefits of a porous, lightweight structure, enabling efficient and sustainable chemical reactions. This essay aims to provide a comprehensive overview of hard foam catalysts, their preparation, properties, and applications.
Preparation of Hard Foam Catalysts
Hard foam catalysts are typically prepared using a foam-templating method. This process involves the creation of a foam, often from a polymeric material, which is then used as a template for the deposition or synthesis of the catalytic material. The foam is subsequently removed, leaving behind a porous, three-dimensional structure with high surface area and excellent mass transport properties.
The choice of foam material and catalyst precursor, as well as the conditions under which the deposition or synthesis occurs, can significantly influence the properties of the resulting hard foam catalyst. For instance, varying the pore size of the foam template can control the size and distribution of the catalytic material, while the use of different precursors can alter the chemical composition and activity of the catalyst.
Properties of Hard Foam Catalysts
Hard foam catalysts exhibit several advantageous properties that make them attractive for various applications. Their high surface area and porosity facilitate efficient contact between the catalyst and reactants, enhancing the rate and yield of chemical reactions. The three-dimensional structure of the catalyst also allows for excellent mass transport, reducing diffusion limitations and improving overall reaction efficiency.
Moreover, hard foam catalysts are typically lightweight and mechanically robust, making them easy to handle and suitable for use in large-scale industrial processes. They can also be designed to exhibit specific chemical properties, such as selectivity towards certain reactions or resistance to deactivation, by carefully controlling the synthesis conditions and choice of catalytic material.
Applications of Hard Foam Catalysts
Hard foam catalysts find applications in a wide range of industries, including chemical manufacturing, environmental remediation, and energy production. In chemical manufacturing, they can be used to catalyze various reactions, such as oxidation, reduction, and hydrogenation, with improved efficiency and selectivity compared to traditional catalysts.
In environmental remediation, hard foam catalysts can be used to degrade pollutants in air and water. Their high surface area and porosity make them effective at adsorbing pollutants, while their catalytic activity enables the degradation of these pollutants into harmless byproducts.
In the energy sector, hard foam catalysts are being explored for use in fuel cells and other energy conversion devices. Their unique structure and properties make them suitable for facilitating the electrochemical reactions that occur in these devices, potentially improving their efficiency and sustainability.
Conclusion
Hard foam catalysts represent a promising development in the field of catalysis, offering a combination of high surface area, porosity, and mechanical robustness that can enhance the efficiency and sustainability of various chemical processes. As our understanding of these materials continues to grow, it is expected that their use will become increasingly widespread, contributing to advancements in chemical manufacturing, environmental remediation, energy production, and beyond.
Recommended Reading:
N-Acetylmorpholine
N-Ethylmorpholine
Morpholine
High Quality 3164-85-0 / K-15 Catalyst / Potassium Isooctanoate
High Quality Bismuth Octoate / 67874-71-9 / Bismuth 2-Ethylhexanoate
Bismuth 2-Ethylhexanoate
Bismuth Octoate
High Quality BDMA / 103-83-3 / Benzyldimethylamine / N,N-dimthylbenzylamine
Benzyldimethylamine
2-(2-Aminoethoxy)ethanol
DMAPA
The Art of Balance: How Balanced Catalysts Optimize Chemical Reactions for Enhanced Efficiency and Sustainability
Explore the world of balanced catalysts and discover how they contribute to greener, more efficient chemical processes across various industries.
Introduction
Catalysts are essential components in many chemical reactions, as they accelerate the reaction rate and reduce the energy required for the process. Balanced catalysts, in particular, have gained significant attention due to their ability to optimize chemical reactions further, leading to enhanced efficiency, selectivity, and sustainability. This article delves into the concept of balanced catalysts, their applications, and the advancements that are shaping their future.
Understanding Balanced Catalysts
Balanced catalysts are designed to maintain a delicate equilibrium between various reaction parameters, such as activity, selectivity, and stability. This balance ensures that the catalyst performs optimally, maximizing the desired product yield while minimizing waste and energy consumption. Balanced catalysts can be achieved through various strategies, including the careful selection of catalyst materials, the modification of their physical and chemical properties, and the optimization of reaction conditions.
Applications of Balanced Catalysts
The versatility of balanced catalysts has led to their widespread adoption across various industries. Some of the most prominent applications include:
-
Petrochemical: Balanced catalysts play a crucial role in the petrochemical industry, where they are used in processes such as hydrocracking, hydrodesulfurization, and reforming. By optimizing these reactions, balanced catalysts contribute to the efficient production of fuels and chemicals with reduced environmental impact.
-
Pharmaceuticals: In the pharmaceutical industry, balanced catalysts are employed to synthesize active pharmaceutical ingredients (APIs) and intermediates. The use of balanced catalysts in these processes ensures high selectivity, minimizing the formation of unwanted by-products and reducing waste generation.
-
Fine Chemicals: The production of fine chemicals, such as flavors, fragrances, and agrochemicals, also benefits from the use of balanced catalysts. These catalysts enable the selective synthesis of complex molecules, leading to improved product quality and reduced energy consumption.
-
Environmental: Balanced catalysts are used in various environmental applications, such as the treatment of exhaust gases and wastewater. By facilitating the efficient removal of pollutants, these catalysts contribute to cleaner and more sustainable industrial processes.
Advancements in Balanced Catalysts Technology
The field of balanced catalysts is continually evolving, with researchers and manufacturers constantly seeking to develop new and improved materials. Some of the latest advancements in balanced catalysts technology include:
-
Nanotechnology: The integration of nanotechnology in balanced catalysts has led to the creation of advanced materials with enhanced properties, such as increased surface area, improved stability, and better dispersion. These features contribute to more efficient and selective catalytic reactions.
-
Computational Design: The use of computational tools, such as density functional theory (DFT) and molecular dynamics simulations, has revolutionized the design of balanced catalysts. These techniques enable researchers to predict the behavior of catalysts under various reaction conditions, facilitating the development of more efficient and sustainable materials.
-
Biocatalysts: Biocatalysts, or enzymes, are a type of balanced catalyst that has gained significant attention due to their unique properties. These naturally occurring catalysts offer high selectivity, mild reaction conditions, and biodegradability, making them an attractive option for greener and more sustainable chemical processes.
The Future of Balanced Catalysts
As the demand for efficient, selective, and environmentally friendly chemical processes continues to grow, the market for balanced catalysts is expected to expand significantly. According to a recent study, the global catalysts market is projected to reach USD 34.2 billion by 2025, growing at a CAGR of 4.2% during the forecast period.
The future of balanced catalysts lies in the development of advanced materials that can address the evolving needs of various industries. Researchers are focusing on creating multifunctional catalysts that can perform multiple reactions simultaneously, leading to more streamlined and efficient processes. Additionally, the development of sustainable and eco-friendly balanced catalysts will continue to be a priority, as the world moves towards a greener and more environmentally conscious future.
Conclusion
Balanced catalysts have undeniably transformed the landscape of chemical reactions, offering unparalleled efficiency, selectivity, and sustainability. As advancements in technology continue to shape the future of balanced catalysts, we can expect to see even more innovative and eco-friendly solutions that will further revolutionize various industries. With their unique properties and wide-ranging applications, balanced catalysts are truly a testament to the power of human ingenuity and the relentless pursuit of progress.
Recommended Reading:
NT CAT ZF-10
NT CAT U28
NT CAT U26
NT CAT K-15
NT CAT D60
TMPEDA
TEDA
Morpholine
2-(2-Aminoethoxy)ethanol
DMAPA
Calculation of catalysts in polyurethane soft foam formulations
The kinetics of the reaction between hydroxyl compounds and isocyanates
-D (- NCO)/dt=K0 x (- NCO) x (- OH)
K1 is the forward reaction rate of the formation of complexes between isocyanates and hydroxyl compounds
K2 is the negative reaction rate of the formation of complexes between isocyanates and hydroxyl compounds
K3 is the forward reaction rate at which complexes react with hydroxyl compounds to form aminoformates and hydroxyl compounds.
K0=[K1 x K3 x (- OH)]/[K2+K3 x
Arrhenius equation
K=Ae ^ [- (Ea/RT)]
A: Exponential factor.
E=2.718
Ea: KJ/mol
R=8.31 (J/mol. K)
Calculation of reaction heat for the formation of functional groups such as urea, polyurethane, biuret, and urea formate:
Bond dissociation energy (KJ/mol)
C-N 205.1~251.2
C-C 230.2~293.0
C-O 293.0-314.0
N-H 351.6~406.0
C-H 364.9~393.5
O-H 422.8~460.5
C=C 418.6~523.3
C=O 594.1~694.9
Reactive equation
RNCO+rOH → RNHCOOr
RNCO+HOH → RNHCOOH+RNCO → RNHCONHR+OCO ↑
RNHCOOr+RNCO → RNCONHRCOOr
RNHCONHr+RNCO → RNCONHRCONHr
The volume ratio of gas to the total volume of the polymerization system (Vg/Vo) in the polymerization system affects the temperature control ability: gas monomers affect the concentration (mol/L), which affects the polymerization heat [Q (KJ/L)=Rp (mol/L) * (- H)]. The heat of polymerization is transferred to the gas dispersion medium, causing the gas to absorb heat and expand (PV=NR/T). After a sudden increase in temperature in the polymerization system, the gas releases and carries away a large amount of heat (approximately in a straight line with Vg/Vo)
When preparing polyurethane in one step, the activation energy of amino acids is about 60 (mol. K), and the activation energy of urea reaction is 17 (mol. K)
The foam system is easier to implement than the solution suspension system. Dispersive polymerization exhibits the Norrish Tromasof effect at the beginning of the reaction, slowing down the rate of change of chain growth parameters over time and improving the monodispersity of the product.
Dispersion polymerization is a method of separating the polymerization system into numerous fine foam by gas, so that the polymerization components can be converted into the surface liquid film of foam and the “polyhedral boundary liquid cell” connecting multiple liquid films can form a special dispersion phase for polymerization.
The foam system uses gas as the dispersion medium, and the gas expands and cools suddenly when it is heated, and the negative pressure generated when the gas escapes will further polymerize the residual single concentration of the system, and accelerate and carry the evaporation of water molecules and the removal of small molecules.
The dispersion effect of gas on the polymerization system is not equivalent to true dilution of monomers.
General formula for half-life of non first-order reactions
T=[2 ^ (n-1) -1]/[a x k x (n-1) x A ^ (n-1)]
Second order reaction rate constant
A+B → Q+S
Kt=[1/(CA0-CB0)] x ln [(CB0 x CA)/(CA0 x CB)]
CA0 x Kt=[1/(1-M)] x ln {[M (1-xA)]/(M-xA)}, where M=CB0/CA0
Attachment:
Example of calculating the density of polyurethane soft foam
Universal polyether ternary alcohol Ppg: 50 pop: 50 tdi-80:42.8 hoh: 3.17 L-580:1 a33:0.34 sn: 0.17
Calculated: 4.34 2.17 6.51 38.2 112% 17% 5.2 1.74 122 Recalculated, 28kg/cubic meter
Example of Calculation for Polyurethane Soft Foam Catalysts
Universal polyether ternary alcohol ppg: 90 pop: 10 tdi-80:: 35.5 hoh: 2.2 L-580:0.84 Black slurry: 6
Calculated: A33:0.18 T-9:0.25
A33:0.14 T-9:0.24
A33:0.13 T-9:0.35
A33:0.12 T-9:0.30
Tolerance and turning points
Calculation of vertical foam flow rate and lifting speed:
Formula (for example only) PPG: 100, TDI: 80, HOH: 6, SI: 1.5, A33: * * *, SN: * * *, MC: 14.8
The diameter of the vertical bubble circular mold is 1.25.
Polyether flow rate is 12 kilograms per minute.
What is the speed of improvement in meters per minute
Calculate the formula density of 12 kilograms per cubic meter. The total weight of the formula is 173.5 kilograms. The formula volume is 14.46 cubic meters. Circular mold cross-sectional area: 1.23 square meters.
Set a loss rate of 5%.
Boosting speed: [14.46 x 12% x (1-5%)]/1.23=1.34 meters per minute.
Related reading recommendations:
Dabco amine catalyst/Low density sponge catalyst
High efficiency amine catalyst/Dabco amine catalyst
Non-emissive polyurethane catalyst/Dabco NE1060 catalyst
Dabco NE1060/Non-emissive polyurethane catalyst
NT CAT A-33
NT CAT A-1
NT CAT 33L
NC CAT T
NT CAT 33LV
3-morpholinopropylamine
4-Acryloylmorpholine
N-Acetylmorpholine
N-Ethylmorpholine
Where isocyanates can be used
Isocyanate is an important organic chemical substance with a wide range of applications in several fields. The following are its main areas of application:
Production of polyurethane: Isocyanate is the main raw material for the production of polyurethane. Through the addition reaction with polyol or polyamine, the hardness, viscosity, density, toughness and other properties of polyurethane can be controlled, so as to prepare polyurethane with different applications. Polyurethane has a wide range of applications in foam, elastomers, coatings, adhesives, cellulose reinforcing materials and other fields.

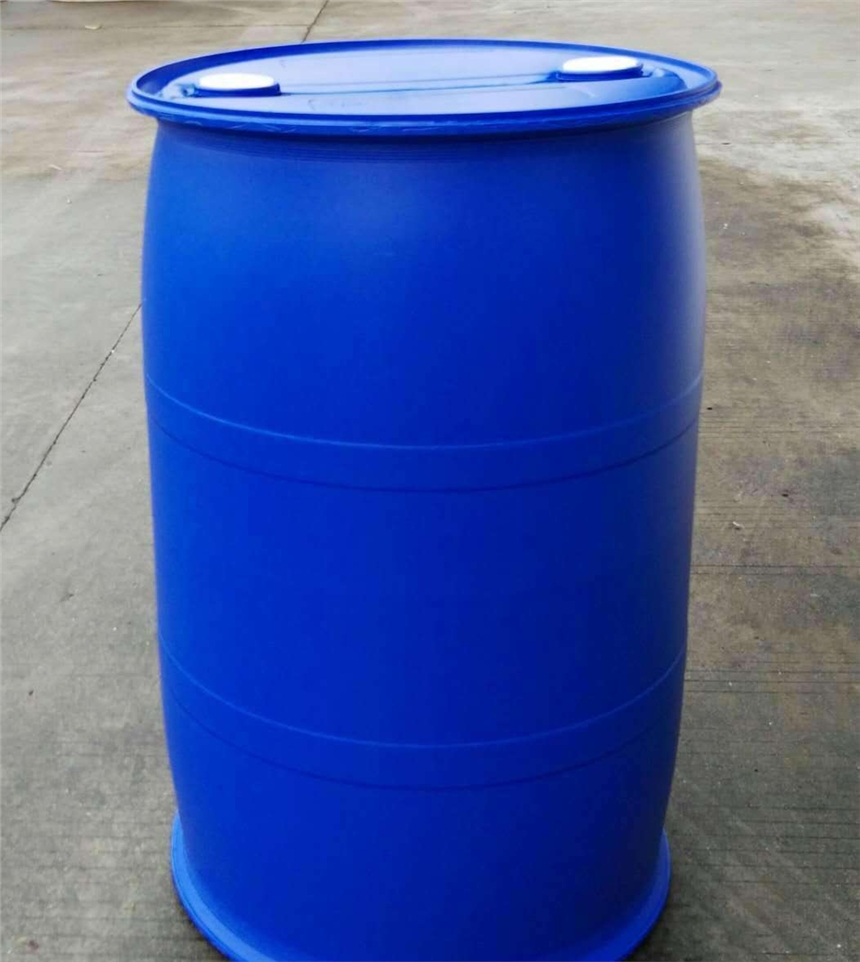
Coatings manufacturing: Isocyanate can be used as a reactive solvent in coatings, reacting with hydroalcohols to generate coating resins, increasing the durability and toughness of coatings and making them more suitable for coatings under various conditions. At the same time, it can also make the adhesion of paint pigments stronger and improve the durability and stain resistance of the paint. This kind of coating is widely used in automotive paints, wood coatings, metal coatings and other fields.
Preparation of adhesives: Isocyanates can be used to produce various types of adhesives, such as water-based polyurethane adhesives, solvent-free adhesives, hot-melt adhesives and so on. These adhesives are widely used in the fields of furniture, shoe materials, automobile interiors, bookbinding and so on.
Biomedical materials: due to the reactivity of isocyanate, it can also be used in the preparation of biodegradable materials and artificial blood vessels, among others. Isocyanates also play an important role in the manufacture of medical equipment and artificial organs.
Other fields: in the printing industry, isocyanate can be used as a component of ink to improve the viscosity, fluidity and adhesion of ink; in the rubber industry, isocyanate can be used as an adhesive; in aquaculture, isocyanate can be used as a water treatment agent to play the role of bactericide and deodorant.
In summary, isocyanates have a wide range of applications in many fields such as polyurethane, coatings, adhesives, printing inks, biomedical materials and so on. However, although isocyanates are widely used, it is still necessary to pay attention to their possible environmental and health impacts in the process of using them, and ensure that they are used in accordance with the relevant safety regulations.
Recommended Related Reading:
Dabco NE1060/Non-emissive polyurethane catalyst
Cyclohexanone – an important intermediate in organic synthesis
Cyclohexanone is a saturated ketone with carbonyl carbon atoms contained within a six membered ring. Although it is cyclic, its properties are similar to those of open chain ketones. It can undergo various reactions such as oxidation, polymerization, and substitution in the presence of a catalyst, and is an important intermediate in organic synthesis.
In the early days, domestic cyclohexanone was only an intermediate product of caprolactam, and the production capacity of manufacturers of cyclohexanone matched that of caprolactam units, with only a small amount of commercial cyclohexanone supplied to the market. The growth and development of cyclohexanone as an independent industry are mainly due to two reasons: firstly, the solvent use of cyclohexanone continues to expand, especially as a high-end organic solvent, it is widely used in industries such as coatings, inks, adhesives, etc., forming a large commodity market; Secondly, with the improvement of domestic production level, cyclohexanone plays an important role in the field of chemical synthesis. In addition to being used for synthesizing caprolactam and adipic acid in the field of chemical fibers, it can also be used to prepare various chemical products such as resins, polycaprolactones, and pharmaceutical intermediates.
Application in the field of chemical synthesis:
1. Caprolactam and Adipic Acid
The main purpose of cyclohexanone is to produce caprolactam and adipic acid, which are important monomers in the production of nylon, nylon 66, and other synthetic resins. In the downstream distribution of cyclohexanone, the chemical fiber industry accounts for over 90% of the total production.
2. Synthetic organic resin
Cyclohexanone can be used to produce cyclohexanone formaldehyde resin, porphyrin resin, aromatic polyamine solid resin, etc. Compared with similar resins, cyclohexanone formaldehyde resin (i.e. ketone formaldehyde resin) has the advantages of high hardness, good weather resistance and oxygen resistance, low viscosity, high glossiness, and compatibility with various paint materials. It is mainly used as a coating resin and is widely used in oil-based resins, alkyd resins, amino resins, acrylic resins, epoxy resins, etc. It can also be used as a dispersant and brightener for inks and ballpoint pen oils. Porphyrin resin has special anti-corrosion properties, which can resist acid corrosion and organic dissolution well, and can be used as an anti-corrosion coating. Aromatic polyamine solid resin can be used as an additive and chain extender for polyurethane rubber.
3. Dehydrogenation to ortho phenylphenol
As an important organic fine chemical product, o-phenylphenol has a wide range of applications and broad prospects. It can be used to synthesize new phosphorus containing flame retardant materials, anti-corrosion and bactericidal agents, printing and dyeing auxiliaries, etc. With further research on o-phenylphenol, its application fields will be wider. The cyclohexanone condensation dehydrogenation method is an ideal production process for preparing o-phenylphenol, with high product purity and wide application range, and has become the mainstream production process.
4. Polycaprolactone
Synthesis of cyclohexanone ε- Caprolactone, ε- Caprolactone is further cyclically polymerized to produce poly (caprolactone) under the action of a catalyst. Polycaprolactone is a white opaque solid with certain rigidity. In addition, it has good biodegradability, drug permeability, and the ability to stably release drugs for a long time. Therefore, polycaprolactone is widely used in drug carriers, coating toughening, biodegradable plastics, polyurethane modification, and other fields.
5. Pharmaceutical intermediates
Cyclohexyl diacetate, abbreviated as CDA, is an important pharmaceutical intermediate prepared from raw materials such as cyclohexanone, acetic acid, and acetic anhydride. It can be used as an intermediate in the production of antiepileptic drugs such as gabapentin.
6. Polyurethane additives
Cyclohexanone can be used to produce polyurethane additive PC8, downstream of which are polyether foaming catalysts, polyurethane foaming catalysts, etc. The terminal includes refrigerator hard foam, board, combination polyether, external wall insulation, insulation layer, etc.
Cyclohexanone is an important organic chemical intermediate with a wide range of applications, involving various fields such as clothing, construction, automotive, pharmaceuticals, packaging, and is closely related to our daily lives. As a major domestic supplier of cyclohexanone, Hualu Hengsheng provides first-class services and stable quality. It has established cooperation with downstream industries and will continue to maintain a stable and high-quality supply of cyclohexanone in the future.
Related reading recommendations:
Tetrachloroethylene Perchloroethylene CAS:127-18-4
DABCO MP608/Delayed equilibrium catalyst
TEDA-L33B/DABCO POLYCAT/Gel catalyst
Addocat 106/TEDA-L33B/DABCO POLYCAT
Understanding Elastomer Catalysts: A Comprehensive Overview
Introduction
Elastomer catalysts play a crucial role in the production of elastomers, a class of polymers characterized by their elasticity and flexibility. These catalysts facilitate the polymerization process, transforming monomers into high molecular weight polymers. This article aims to provide a comprehensive overview of elastomer catalysts, their types, functions, and applications.
Types of Elastomer Catalysts
Elastomer catalysts can be broadly categorized into two types: peroxide catalysts and metallic catalysts.
Peroxide Catalysts: These are organic compounds containing two oxygen atoms linked together (-O-O-). They are capable of initiating polymerization by decomposing to form free radicals. Commonly used peroxide catalysts include dicumyl peroxide, benzoyl peroxide, and tert-butyl hydroperoxide.
Metallic Catalysts: These are typically transition metal compounds that can initiate polymerization through coordination or insertion mechanisms. Examples include titanium, zirconium, and lanthanide compounds.
Functions of Elastomer Catalysts
The primary function of elastomer catalysts is to initiate the polymerization process. They do this by providing active sites for monomer molecules to attach and grow into polymer chains. The choice of catalyst can significantly influence the properties of the resulting elastomer, including its molecular weight, polydispersity, and tacticity.
Applications of Elastomer Catalysts
Elastomer catalysts are used in a wide range of industries due to the versatile properties of elastomers. Some common applications include:
Automotive Industry: Elastomers are used in the production of tires, hoses, seals, and belts. The catalysts used in these applications need to provide elastomers with excellent heat resistance, durability, and flexibility.
Construction Industry: Elastomers are used in roofing membranes, sealants, and insulation materials. The catalysts used here need to provide elastomers with good weather resistance and long-term stability.
Medical Industry: Elastomers are used in the production of medical devices, such as catheters, tubing, and syringes. The catalysts used in these applications need to provide elastomers with excellent biocompatibility and sterilization resistance.
Conclusion
Elastomer catalysts are essential components in the production of elastomers, influencing their properties and determining their suitability for various applications. Understanding the types, functions, and applications of these catalysts can help in the development of new elastomers with improved properties and performance. As research continues in this field, we can expect to see advancements in elastomer catalysts, leading to the production of elastomers with enhanced properties and broader applications.