Skip to content
Introduction
Greenhouse gas (GHG) emissions from chemical manufacturing processes pose a significant challenge in the global fight against climate change. These emissions not only contribute to global warming but also impact air quality and public health. In response to these concerns, scientists and researchers are continuously seeking innovative solutions to reduce the environmental footprint of chemical manufacturing. A recent breakthrough in the development of a new chemical catalyst promises to significantly minimize GHG emissions, paving the way for more sustainable industrial processes.
The Catalyst Breakthrough
A team of researchers from a renowned international research institute has developed a novel chemical catalyst that can substantially reduce GHG emissions in chemical manufacturing processes. The groundbreaking catalyst, composed of earth-abundant materials, facilitates chemical reactions more efficiently, leading to lower energy consumption and reduced emissions of harmful gases, such as carbon dioxide (CO2) and nitrous oxide (N2O).
The new catalyst is designed to replace traditional catalysts that rely on precious metals, such as platinum and palladium, which are both expensive and limited in supply. By utilizing earth-abundant materials, the novel catalyst offers a more cost-effective and sustainable solution for chemical manufacturing.
Impact on Chemical Manufacturing Processes
The innovative catalyst has the potential to revolutionize various chemical manufacturing processes, including the production of pharmaceuticals, agrochemicals, and polymers. By enhancing the efficiency of chemical reactions, the catalyst can lead to substantial energy savings and reduced GHG emissions in these industries.

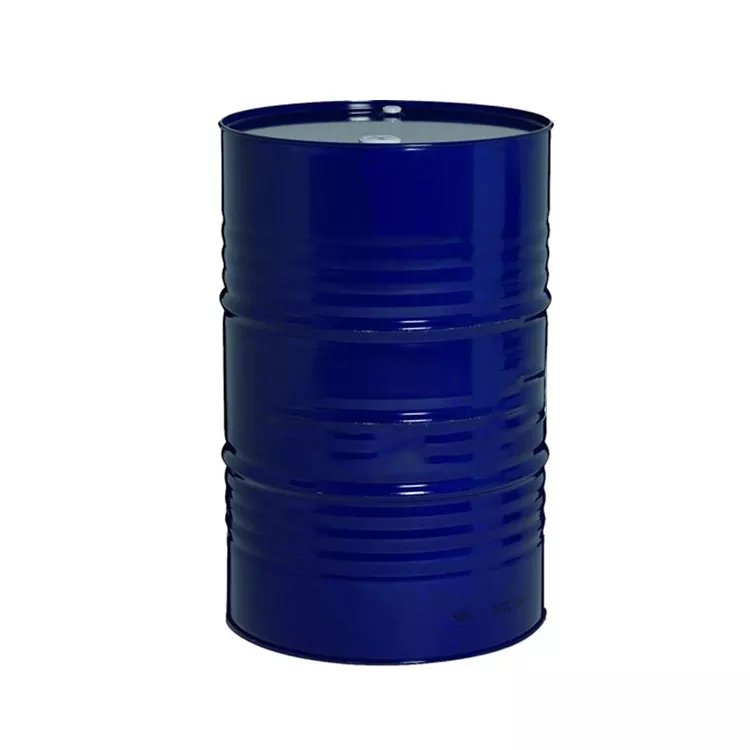
For instance, in the production of pharmaceuticals, the new catalyst can facilitate the selective synthesis of active pharmaceutical ingredients (APIs), minimizing the generation of waste and by-products. This not only reduces the environmental impact of pharmaceutical manufacturing but also improves the overall yield and cost-effectiveness of the process.
Similarly, in the production of agrochemicals, the novel catalyst can promote the formation of desired chemical compounds while minimizing the emission of harmful gases. This can contribute to cleaner and more sustainable agricultural practices, ultimately benefiting both the environment and human health.
Moreover, the new catalyst can also play a significant role in the production of polymers, which are widely used in various industries, including packaging, automotive, and construction. By facilitating the efficient polymerization of raw materials, the catalyst can help reduce energy consumption and GHG emissions in polymer manufacturing, leading to a more sustainable plastics industry.
Environmental and Economic Benefits
The adoption of the new chemical catalyst in industrial processes offers numerous environmental and economic benefits. By reducing GHG emissions, the catalyst can help mitigate climate change and improve air quality, contributing to a healthier and more sustainable planet.
Furthermore, the use of earth-abundant materials in the catalyst’s composition makes it a more cost-effective solution compared to traditional catalysts that rely on precious metals. This can lead to significant cost savings for chemical manufacturers, making the industry more competitive and resilient.
Additionally, the novel catalyst’s ability to improve the efficiency of chemical reactions can result in higher yields and lower waste generation, further enhancing the economic viability of chemical manufacturing processes.
Conclusion
The development of the new chemical catalyst represents a significant milestone in the quest for more sustainable and environmentally friendly industrial processes. By reducing greenhouse gas emissions and improving the efficiency of chemical reactions, the innovative catalyst offers a promising solution for addressing the challenges associated with chemical manufacturing. As research and development in this area continue to advance, it is expected that the new catalyst will play an increasingly important role in shaping the future of the chemical industry, contributing to a greener and more sustainable world.
Recommended reading:
Introduction
Batteries have become an integral part of our daily lives, powering various devices ranging from smartphones and laptops to electric vehicles (EVs) and renewable energy storage systems. As the demand for efficient, long-lasting, and eco-friendly batteries continues to grow, researchers are exploring innovative ways to improve battery performance and lifespan. One promising approach involves the use of chemical catalysts, which can enhance the electrochemical reactions within batteries, leading to better efficiency, faster charging times, and extended lifespan. This essay will discuss the role of chemical catalysts in improving battery performance and life, focusing on various types of batteries and catalyst materials.
Lithium-ion Batteries
Lithium-ion batteries (LIBs) are currently the most widely used rechargeable batteries due to their high energy density, long cycle life, and low self-discharge rate. However, there is still room for improvement, particularly in terms of charging speed, safety, and environmental impact. Chemical catalysts can play a crucial role in addressing these challenges.
One way catalysts can improve LIB performance is by enhancing the lithium-ion intercalation/deintercalation process, which occurs during charging and discharging. Transition metal oxides, such as manganese oxide (MnO2) and cobalt oxide (Co3O4), have been shown to be effective catalysts for facilitating this process, leading to faster charging times and improved energy density.
Another area where catalysts can make a significant impact is in the development of solid-state lithium batteries, which use a solid electrolyte instead of a liquid one. Solid-state batteries offer several advantages, including increased safety, higher energy density, and a longer lifespan. However, the challenge lies in finding suitable catalyst materials that can facilitate lithium-ion transport through the solid electrolyte. Researchers have identified several promising catalysts, such as lithium nitride (Li3N) and lithium phosphorus oxynitride (LiPON), which can enhance ionic conductivity and improve overall battery performance.

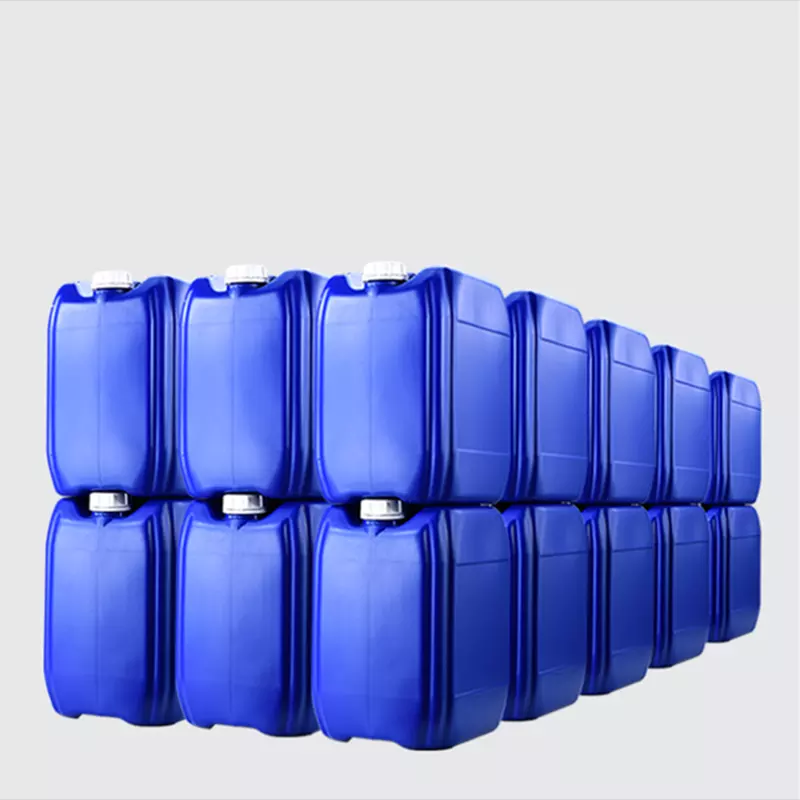
Redox Flow Batteries
Redox flow batteries (RFBs) are a type of rechargeable battery that stores energy in liquid electrolytes containing redox-active species. RFBs have several advantages, including scalability, long cycle life, and the ability to decouple energy and power capacities. However, they also face challenges related to energy density, efficiency, and the cost of electrolyte materials.
Chemical catalysts can help address these challenges by facilitating the redox reactions that occur at the electrodes during charging and discharging. For example, in vanadium redox flow batteries (VRFBs), which use vanadium ions in different oxidation states as the active species, researchers have developed catalysts based on transition metal carbides and nitrides to enhance the electrochemical reactions and improve overall battery performance.
Similarly, in zinc-air batteries, which rely on the oxidation of zinc and the reduction of oxygen from the air, chemical catalysts can play a critical role in enhancing the oxygen reduction reaction (ORR) and oxygen evolution reaction (OER). Noble metal catalysts, such as platinum and iridium, have shown excellent catalytic activity for these reactions. However, their high cost and limited availability have prompted researchers to explore alternative materials, such as transition metal oxides, sulfides, and nitrides, which offer comparable performance at a lower cost.
Sodium-ion Batteries
Sodium-ion batteries (SIBs) are an emerging alternative to LIBs, as they utilize abundant and low-cost sodium instead of lithium. However, SIBs face challenges related to energy density, cycle life, and electrode material stability. Chemical catalysts can help overcome these challenges by enhancing the electrochemical reactions and improving the performance of electrode materials.
For instance, researchers have developed various catalyst materials, such as transition metal oxides and phosphates, to facilitate sodium-ion intercalation/deintercalation in cathode materials, leading to improved energy density and cycle life. Additionally, catalysts can help stabilize the solid electrolyte interphase (SEI) layer, which forms on the anode surface during battery operation, thereby enhancing the overall stability and lifespan of SIBs.
Conclusion
Chemical catalysts play a crucial role in improving battery performance and lifespan, offering the potential to address the challenges associated with various types of batteries, including LIBs, RFBs, and SIBs. By facilitating electrochemical reactions, enhancing ion transport, and stabilizing electrode materials, catalysts can contribute to the development of more efficient, long-lasting, and eco-friendly batteries. As research in this area continues to advance, it is expected that chemical catalysts will become an increasingly important component in the design and optimization of next-generation battery systems.
Recommended reading: