Author: admin
What are the ingredients contained in the varnish `hardener` thinner?
Diluent is based on Cyclohexanone. Butyl acetate. Anhydrous xylene is a mixture of organic solvents.
Curing agents, also known as hardeners, curing agents or modifiers, are a class of substances or mixtures that enhance or control the curing reaction.
Resin curing is the process of irreversible changes in thermosetting resins through chemical reactions such as condensation, ring closure, addition or catalysis, and curing is accomplished by the addition of curing (cross-linking) agents.
Classification of epoxy resin curing agents
(1) Alkaline and acidic curing agents:
(1) alkaline curing agents, including aliphatic diamines and polyamines, aromatic polyamines, other nitrogen compounds and modified fatty amines.
② acidic curing agents, including organic acids, anhydrides, and boron trifluoride and its complexes.
(2) Forming and catalytic curing agents:
This kind of curing agent and epoxy group undergoes addition reaction to form part of the chain segment of the curing product, and makes the linear molecule crosslinked into the body structure molecule through gradual polymerisation reaction, and this kind of curing agent is also known as melon-type curing agent. Such as primary amines, polythiols, polyphenols, low molecular polyamides, organic acids and anhydrides and low condensate curing agent.
② catalytic curing agent This type of curing agent only on the epoxy resin to trigger the role of opening the epoxy group, catalytic epoxy resin itself polymerisation into a network structure, the generation of ether bond as the main structure of the homopolymer, and the curing agent itself does not produce crosslinking reaction. Such as tertiary amine, imidazole, dicyandiamine, boron trifluoride complex, stannous chloride, stannous isooctanoate and stannous octanoate.

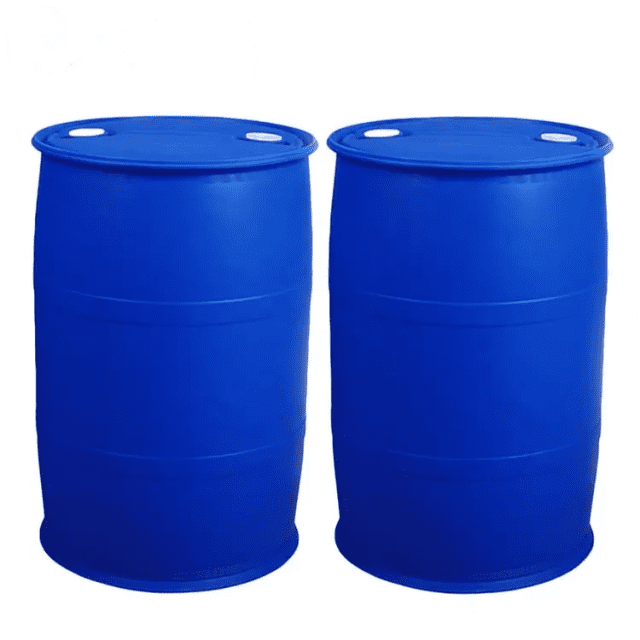
Common epoxy resin curing agent introduction:
Epoxy resin curing material has excellent mechanical properties, electrical properties, chemical resistance, and therefore widely used. Curing agent is one of the necessary raw materials for epoxy resin curing material, otherwise the epoxy resin will not be cured. In order to meet the requirements of various applications, the corresponding curing agent should be used. There are many types of curing agents, which are introduced below:
Fatty polyamines
Ethylenediamine
EDA H2NCH2CH2NH2 Molecular Weight 60 Active Hydrogen Equivalent 15 Colourless Liquid 6-8 parts per 100 parts of Standard Resin
Properties: toxic, aggressive odour, high volatility, low viscosity, fast curing at room temperature. Used for bonding, casting and coating. This kind of amine increases with molecular weight, viscosity increases, volatility decreases, toxicity decreases and performance improves. However, they have high heat release and short application period. Generally speaking, the higher molecular weight they have, the less they are affected by the amount of coordination. Prolonged exposure to fatty polyamines can cause dermatitis, and their vapour toxicity is very strong, so they should be operated with great care.
Diethylenetriamine
DETA H2NC2H4NHC2H4NH2 Molecular Weight 103 Active Hydrogen Equivalent 20.6 Colourless Liquid
Use 8-11 parts per 100 parts of standard resin. Curing: 2 hours at 20°C + 30 minutes at 100°C or 4 days at 20°C. Properties: Applicable period 50g 25°C for 45 minutes, heat distortion temperature 95-124°C, flexural strength 1000-1160kg/cm2, compressive strength 1120kg/cm2, tensile strength 780kg/cm2, elongation 5.5%, impact strength
0.4 ft-lb/in Rockwell hardness 99-108. dielectric constant (50 Hz, 23°C) 4.1 power factor (50 Hz, 23°C) 0.009 bulk resistance 2×1016
Ω-cm Curing at room temperature, high toxicity, high heat release, short application period.
Triethylenetetramine TETA H2NC2H4NHC2H4NHC2H4NH2
Molecular weight 146 Active hydrogen equivalent 24.3 Colourless viscous liquid 10-13 parts per 100 parts of standard resin
Curing: 2 hours at 20°C + 30 minutes at 100°C or 7 days at 20°C. Properties: Applicable period 50g 25℃45 minutes, heat distortion temperature 98-124℃, flexural strength 950-1200kg/cm2, compressive strength 1100kg/cm2, tensile strength 780kg/cm2, elongation 4.4%, impact strength
0.4 ft-lb/in Rockwell hardness 99-106, room temperature curing, toxicity is slightly lower than diethylenetriamine, heat release, short application period.
Tetraethylenepentamine TEPA
H2NC2H4(NHC2H4)3NH2 Molecular Weight 189 Active Hydrogen Equivalent 27 Brown Liquid 11-15 parts per 100 parts of standard resin.
Properties as above.
PEPA H2NC2H4(NHC2H4)nNH2 Light Yellow Liquid 14-15 parts per 100 parts of standard resin.
Properties: low toxicity, low volatility, long application period, low price.
Dipropylenetriamine DPTA H2N(CH2)3 NH(CH2)3NH2
Molecular weight 131 Active hydrogen equivalent 2
Recommended Related Reading:
Dabco NE1060/Non-emissive polyurethane catalyst
Non-emissive polyurethane catalyst/Dabco NE1060 catalyst
DMAPA
2-(2-Aminoethoxy)ethanol
Morpholine
TEDA
Jeffcat ZF-22
BDMAEE Exporter
N-Methylmorpholine
4-Formylmorpholine
Chilling Innovations: How Refrigerator Catalysts Enhance Energy Efficiency and Air Quality for a Greener Future
Explore the world of refrigerator catalysts and discover how they contribute to improved energy efficiency, air purification, and environmental sustainability in modern refrigeration systems.
Introduction
Refrigerator catalysts are specialized materials designed to improve the performance and energy efficiency of refrigeration systems while also enhancing air quality. These innovative catalysts play a crucial role in promoting a greener and more sustainable future for refrigeration technology. This article delves into the concept of refrigerator catalysts, their applications, and the advancements that are shaping their future.
Understanding Refrigerator Catalysts
Refrigerator catalysts are typically composed of metal or metal oxide nanoparticles, which are integrated into various components of the refrigeration system, such as the evaporator, condenser, or air filters. They function by facilitating chemical reactions that help to eliminate volatile organic compounds (VOCs), bacteria, and other airborne contaminants, resulting in improved air quality and reduced energy consumption.
Applications of Refrigerator Catalysts
The versatility of refrigerator catalysts has led to their widespread adoption across various refrigeration systems. Some of the most prominent applications include:
Air Purification: Refrigerator catalysts are used in air filters to remove VOCs, bacteria, and other airborne contaminants, ensuring that the air inside the refrigerator remains fresh and clean. This not only enhances food safety but also reduces the need for frequent cleaning and maintenance.
Energy Efficiency: Refrigerator catalysts can improve the heat transfer efficiency of the evaporator and condenser coils, leading to reduced energy consumption and lower operating costs. By facilitating the breakdown of frost and ice buildup, these catalysts also help to maintain optimal cooling performance.
Odor Elimination: Refrigerator catalysts can effectively eliminate unpleasant odors caused by food spoilage or other sources. This is achieved through the oxidation of odor-causing molecules, which are converted into harmless by-products such as water and carbon dioxide.
Advancements in Refrigerator Catalysts Technology
The field of refrigerator catalysts is continually evolving, with researchers and manufacturers constantly seeking to develop new and improved materials. Some of the latest advancements in refrigerator catalysts technology include:
Nanotechnology: The integration of nanotechnology in refrigerator catalysts has led to the creation of advanced materials with enhanced properties, such as increased surface area, improved stability, and better dispersion. These features contribute to more efficient and effective air purification and energy savings.
Photocatalysts: Photocatalysts are a type of refrigerator catalyst that utilizes light energy, typically from UV or visible light, to initiate chemical reactions. These materials can effectively decompose organic pollutants and eliminate bacteria, making them an attractive option for air purification and odor elimination in refrigeration systems.
Multifunctional Catalysts: Researchers are focusing on developing multifunctional refrigerator catalysts that can perform multiple tasks simultaneously, such as air purification, odor elimination, and energy efficiency enhancement. These innovative materials have the potential to revolutionize the refrigeration industry by offering comprehensive performance improvements in a single solution.
The Future of Refrigerator Catalysts
As the demand for energy-efficient, eco-friendly, and high-performance refrigeration systems continues to grow, the market for refrigerator catalysts is expected to expand significantly. According to a recent study, the global refrigerator catalysts market is projected to reach USD 1.2 billion by 2026, growing at a CAGR of 6.8% during the forecast period.
The future of refrigerator catalysts lies in the development of advanced materials that can address the evolving needs of the refrigeration industry. Researchers are focusing on creating more efficient, durable, and sustainable catalysts that can contribute to greener and more environmentally conscious refrigeration systems.
Conclusion
Refrigerator catalysts have undeniably transformed the landscape of refrigeration technology, offering unparalleled energy efficiency, air purification, and sustainability. As advancements in technology continue to shape the future of refrigerator catalysts, we can expect to see even more innovative and eco-friendly solutions that will further revolutionize the refrigeration industry. With their unique properties and wide-ranging applications, refrigerator catalysts are truly a testament to the power of human ingenuity and the relentless pursuit of progress.
Recommended Reading:
NT CAT DMDEE
NT CAT PC-5
NT CAT DMP-30
NT CAT DMEA
NT CAT BDMA
NT CAT PC-9
NT CAT ZR-50
NT CAT TMR-2
NT CAT PC-77
NT CAT PC-41
NT CAT PC-8
What are all the catalysts in the synthesis of polylactic acid?
The catalysts used in the synthesis of PLA are mainly as follows:
Tin catalysts: This is a class of catalysts commonly used in the synthesis of PLA, including tin, stannous chloride and stannous octanoate. Among them, stannous octanoate is considered to be the best catalyst. Under certain conditions, PLA synthesised using stannous octanoate as catalyst has excellent properties, such as no oxidation, discolouration, and the viscosity average molecular weight can reach a high level.
Metal catalysts: Metal catalysts also play an important role in the synthesis of PLA. These catalysts can promote the condensation reaction of lactic acid monomers, so as to effectively form PLA chains.
In addition to the common catalysts mentioned above, there are some other types of catalysts that may be used in the synthesis of PLA, but the exact catalyst to be used depends on factors such as the synthesis method, the reaction conditions, and the properties of the desired PLA. Therefore, when selecting a catalyst, various factors need to be considered in order to achieve the best synthesis results.
Please note that the selection and use of catalysts should follow the relevant safety regulations and operating procedures to ensure the safety and reliability of the experimental process. Meanwhile, with the continuous development of science and technology, new types of catalysts are emerging, so it may also be necessary to pay attention to the latest research results and technological advances in practical applications.
Recommended Related Reading:
Dabco NE1060/Non-emissive polyurethane catalyst
How to prepare a solution of stannous toluene octanoate
To prepare the stannous octanoate toluene solution, the following steps may be followed:
Prepare the required materials and equipment including stannous octanoate, toluene solvent, containers, stirrers and suitable heating equipment.
Take an appropriate amount of the toluene solvent and pour it into the container and heat it to a suitable temperature to induce the dissolution process. The exact heating temperature should be determined according to the nature of the toluene solvent and stannous octanoate used to ensure that the temperature is not too high to cause volatilisation of the solvent or other unfavourable reactions.
Gradually add the corresponding proportion of stannous octanoate while mixing well with a stirrer. The amount of stannous octanoate added should be determined by the desired concentration of the solution.
Stir continuously until the stannous octanoate is completely dissolved in the toluene solvent and a homogeneous solution is formed.
Please note that the preparation process should be carried out in a safe manner, avoiding contact with skin and inhalation of harmful gases. Meanwhile, both stannous octanoate and toluene are chemical substances that need to be stored and used under suitable conditions to avoid reaction with other substances or causing environmental pollution.
In addition, the specific proportion and conditions of preparation may vary depending on the application requirements, nature of the materials or safety regulations. Therefore, in practice, it is recommended to refer to relevant chemical manuals, professional information or consult chemical experts to ensure the accuracy and safety of the formulation process.
Recommended Related Reading:
Dabco NE1060/Non-emissive polyurethane catalyst
Calculation of catalysts in polyurethane soft foam formulations
The kinetics of the reaction between hydroxyl compounds and isocyanates – d (- NCO)/dt=K0 x (- NCO) x (- OH)
K1 is the forward reaction rate of the formation of complexes between isocyanates and hydroxyl compounds
K2 is the negative reaction rate of the formation of complexes between isocyanates and hydroxyl compounds
K3 is the forward reaction rate at which complexes react with hydroxyl compounds to form aminoformates and hydroxyl compounds.
K0=[K1 x K3 x (- OH)]/[K2+K3 x
Arrhenius equation
K=Ae ^ [- (Ea/RT)]
A: Exponential factor.
E=2.718
Ea: KJ/mol
R=8.31 (J/mol. K)
Calculation of reaction heat for the formation of functional groups such as urea, polyurethane, biuret, and urea formate:
Bond dissociation energy (KJ/mol)
C-N 205.1~251.2
C-C 230.2~293.0
C-O 293.0-314.0
N-H 351.6~406.0
C-H 364.9~393.5
O-H 422.8~460.5
C=C 418.6~523.3
C=O 594.1~694.9
Reaction formula:
RNCO+rOH → RNHCOOr
RNCO+HOH → RNHCOOH+RNCO → RNHCONHR+OCO ↑
RNHCOOr+RNCO → RNCONHRCOOr
RNHCONHr+RNCO → RNCONHRCONHr
The volume ratio of gas to the total volume of the polymerization system (Vg/Vo) in the polymerization system affects the temperature control ability: gas monomers affect the concentration (mol/L), which affects the polymerization heat [Q (KJ/L)=Rp (mol/L) * (- H)]. The heat of polymerization is transferred to the gas dispersion medium, causing the gas to absorb heat and expand (PV=NR/T). After a sudden increase in temperature in the polymerization system, the gas releases and carries away a large amount of heat (approximately in a straight line with Vg/Vo)
When preparing polyurethane in one step, the activation energy of amino acids is about 60 (mol. K), and the activation energy of urea reaction is 17 (mol. K)
The foam system is easier to implement than the solution suspension system. Dispersive polymerization exhibits the Norrish Tromasof effect at the beginning of the reaction, slowing down the rate of change of chain growth parameters over time and improving the monodispersity of the product.
Dispersion polymerization is a method of separating the polymerization system into numerous fine foam by gas, so that the polymerization components can be converted into the surface liquid film of foam and the “polyhedral boundary liquid cell” connecting multiple liquid films can form a special dispersion phase for polymerization.
The foam system uses gas as the dispersion medium, and the gas expands and cools suddenly when it is heated, and the negative pressure generated when the gas escapes will further polymerize the residual single concentration of the system, and accelerate and carry the evaporation of water molecules and the removal of small molecules.
The dispersion effect of gas on the polymerization system is not equivalent to true dilution of monomers.
General formula for half-life of non first-order reactions
T=[2 ^ (n-1) -1]/[a x k x (n-1) x A ^ (n-1)]
Second order reaction rate constant
A+B → Q+S
Kt=[1/(CA0-CB0)] x ln [(CB0 x CA)/(CA0 x CB)]
CA0 x Kt=[1/(1-M)] x ln {[M (1-xA)]/(M-xA)}, where M=CB0/CA0
Attachment:
Example of calculating the density of polyurethane soft foam
General polyether ternary alcohol Ppg: 50pop: 50tdi-80:42.8hoh: 3.17L-580:1a33:0.34sn: 0.17
Calculated: 4.34 2.17 6.51 38.2 112% 17% 5.2 1.74 122 Recalculated, 28kg/cubic meter
Example of calculation for polyurethane soft foam catalyst:
Universal polyether ternary alcohol ppg: 90 pop: 10 tdi-80:: 35.5 hoh: 2.2 L-580:0.84 Black slurry: 6
Calculated: A33:0.18 T-9:0.25
A33:0.14 T-9:0.24
A33:0.13 T-9:0.35
A33:0.12 T-9:0.30
Tolerance and turning points
Calculation of vertical foam flow rate and lifting speed:
Formula (for example only) PPG: 100, TDI: 80, HOH: 6, SI: 1.5, A33: * * *, SN: * * *, MC: 14.8
The diameter of the vertical bubble circular mold is 1.25.
Polyether flow rate is 12 kilograms per minute.
What is the speed of improvement in meters per minute
Calculate the formula density of 12 kilograms per cubic meter. The total weight of the formula is 173.5 kilograms. The formula volume is 14.46 cubic meters. Circular mold cross-sectional area: 1.23 square meters.
Set a loss rate of 5%.
Boosting speed: [14.46 x 12% x (1-5%)]/1.23=1.34 meters per minute.
Related reading recommendations: